|
Variety development |
Since the dawn of agriculture some 10,000 years ago, farmers have been developing varieties of plants to yield desired results.
|
Introduction |
Since the dawn of agriculture some 10,000 years ago, farmers have been developing varieties of plants to yield desired results. When you eat an ear of corn, for example, you can thank the prehistoric agriculturalists who started the process by selecting types of maize that retained their seed on the ear, leading to the development of corn on the cob. Variety development can be accomplished through many activities, from simply selecting plants with desirable characteristics for propagation to more complex molecular breeding techniques.
Plant breeding is the generation of variation, selection of plants and genetic stabilization (fixing) of traits to obtain varieties with reproducible desired characteristics. Its scientific underpinnings began to be understood in the mid-nineteenth century with the work of Gregor Mendel, the father of genetics. Using the pea plants in his garden, Mendel observed how traits were passed down to succeeding generations and he formulated the idea that specific traits were inherited as units in a predictable way. By the mid-twentieth century, scientists had established that traits are transmitted by genes in chromosomes, which store and express chemical information resulting in these characteristics. An understanding of genetic principles and their application to plant breeding technology has greatly accelerated the rate of improvement of crop plants. Researchers estimate that at least half of the several-fold yield increases attained in wheat and rice during the Green Revolution* resulted from the development and use of genetically improved varieties.
Plant traits are encoded in the DNA of their genes. Sometimes many different genes can influence a desirable trait, making it difficult for plant breeders to accumulate them all into a single variety. Marker-assisted breeding allows breeders to map and trace thousands of genes and screen large populations of plants for those that possess the traits of interest. The marker, or genetic tag, can be based on either DNA or proteins. Molecular markers have enabled high-throughput genotyping and accelerated the rate at which breeders can incorporate useful traits into new varieties.
Breeders often make crosses between plants of diverse genetic makeup or genotypes* to produce new combinations of genetic traits, which then result in diverse phenotypes*, or observable morphological or quality traits in the progeny plants. The natural diversity of different sources of germplasm within a species or its close relatives is a primary source of genetic variation. Genetic variation can also be increased by inducing mutations, changes in the DNA sequences of the plants. Since the 1950s, over 2,200 crop varieties have been developed by induced mutations. In 1973, it became possible to identify and splice (or recombine) specific DNA molecules, leading to recombinant DNA technology or genetic engineering, which allows scientists to copy and exchange genes among species to introduce new characteristics, such as resistance to herbicides (compounds that control weeds) or insects. Plants developed using genetic engineering are often called transgenic plants.
Green Revolution |
Advances in genetics, crop protection, fertilizers and machinery that culminated in dramatic increases in crop productivity during the third quarter of the 20th century. |
Genotype |
the total of all genetic information contained in an organism, regardless of whether it is evident in the observable or measureable traits (the phenotype). |
Phenotypes |
Observable or measureable characteristics of an organism that result from interactions of its genetic constitution (its genotype) with the environment in which it grows. |
|
|
Plant Breeding |
Plant breeding is an ancient art and modern science. From the earliest origins of agriculture, people consciously or subconsciously saved seeds from plants with desirable traits in a natural population* and used them to plant the next generation, a process called selection.
Gradually, after a number of generations of repeated selection, most of the plants in the population will have the desired traits.
For this to be effective, there must be genetic variation* for the traits being selected. Genetic variation can be obtained by identifying naturally varying types and by making controlled sexual crosses by transferring pollen* from one plant to the pistil* of another to create seeds carrying traits (controlled by genes*) from two or many parents.
The progeny* seeds can be planted and the resulting plants carrying the desired traits from both parents can be selected. In this manner, the desirable traits from two or more plants can be combined into single plants which can be further selected and propagated*. Plant breeding is the process of repeatedly sampling or generating genetic variation, selecting desirable plants, fixing the traits and propagating plant materials.
Our understanding of genetic inheritance* at the whole organism and cellular* levels has allowed us to create the vast diversity of foods, feed and fiber crops grown today.
Natural population |
a population of plants growing in the wild |
Genes |
units of inheritance that are encoded in an organism’s DNA |
Genetic variation |
differences for a trait that are passed from one generation to another |
Pistil |
the female part of a flower that grows into a fruit containing seeds. |
Pollen |
structures that carry the male genetic material and fertilize the egg cells to create embryos inside seeds |
Progeny |
the descendants of a plant |
Propagate |
to reproduce |
Genetic inheritance |
the transfer of traits from female and male parents to their descendants |
Cell |
the fundamental building block of living tissues |
|
|
Genetic Engineering |
Plant traits are encoded in the DNA of their genes. In 1973 it became possible to identify and splice, or recombine, specific DNA molecules, leading to recombinant DNA technology, or genetic engineering, which allows scientists to copy and exchange genes among organisms to introduce new characteristics, such as resistance to herbicides or insects. Plants developed using genetic engineering are often called transgenic plants.
Although genetic engineering of foods was commercialized first in yeast to produce rennet for making cheese in the late 1980s in Europe, the first commercial genetically engineered crop was the FLAVR SAVR tomato from Calgene Inc., first sold in 1994. The first large-scale introduction of a transgenic crop occurred in 1995 with the release of herbicide-tolerant soybeans.
Along with insect resistance conferred by the Bacillus thuringiensis (Bt) protein, this first generation of genetically engineered crops exhibited improved agronomic traits.
that 68 percent of soybeans, 26 percent of corn and 69 percent of cotton in the United States were planted with varieties enhanced by genetic engineering.
Worldwide, 130 million acres of transgenic crops were grown in 2001. Genetically engineered crops have continued to increase 10-15% annually, with over 300 million acres (120 M ha) grown in 25 countries in 2008 (ISAAA 2009).
The second generation of transgenic crops to enter the market is expected to incorporate many value-enhanced qualities, such as higher nutrient contents, increased fertilizer use efficiency and resistance to environmental stresses such drought.
One example of quality enhancement is Golden Rice®, a rice variety that accumulates beta-carotene (the precursor to vitamin A) and iron in the grain, potentially reducing childhood blindness and anemia in countries where rice is a staple food.
|
Genotyping |
An organism’s specific genomic complement, or the DNA sequences of all of its genes and intervening DNA regions, constitutes its genotype.
Most genes are present in a species in a number of different variants, or alleles, which may or may not affect the function of the gene.
By scoring genetic locations within an individual’s genome to know which alleles it has inherited from its parents, the specific genotype can be identified.
For example, if a particular gene confers resistance to a disease, seedling plants can be screened for the presence of this gene and only those with the resistant allele of the gene can be grown for further testing and propagation.
Such early genotyping and selection can result in large savings in labor, land and materials when screening large populations, particularly when alternative phenotypic screens, such as infecting all of the plants with the disease organism, are labor-intensive and expensive.
High-throughput methods enable the simultaneous testing of thousands of genes in hundreds of individuals, making it feasible to select for multiple traits in large plant populations.
|
Induced Mutation |
A mutation is any change in a DNA sequence that can be passed from parent to offspring. By inducing mutations, scientists have been able to increase genetic variation, which breeders depend on to produce crops with desirable traits, such as resistance to diseases and insects.
Unlike recombinant DNA techniques, induced mutation does not add any foreign genetic material into the plant.
Essentially, it produces results that could have occurred through naturally occurring mutations and selection of desirable progeny. However, as mutations occur naturally at low frequencies and randomly in the DNA, it would require much more time to achieve such results.
Mutations can be induced in a variety of ways, such as by exposure to ultraviolet or ionizing radiation or chemical mutagens.
Since the 1950s, over 2,000 crop varieties have been developed by inducing mutations to randomly alter genetic traits and then selecting for improved types among the progeny.
Many common foods, such as popular red grapefruit varieties, are the result of induced mutations. Recently, methods have been developed to allow scientists to efficiently identify and select for mutations in specific genes.
This is enhancing the utilization of induced mutation in plant breeding, as changes in genes known to produce specific results can be identified rather than having to screen very large populations of plant to find the rare individuals with the desired trait.
|
Marker-assisted breeding |
Marker-assisted (or molecular-assisted) breeding is the use molecular markers to track the genetic makeup of plants during the variety development process. It provides a dramatic improvement in the efficiency with which breeders can select plants with desirable combination of genes.
A molecular marker is a genetic tag that identifies a particular location within a plant’s DNA sequences.
Markers can be used in transferring a single gene into a new cultivar or in testing plants for the inheritance for many genes at once. Markers can be based on either DNA or proteins.
Both DNA- and protein-based markers have been widely used in plant breeding, but DNA-based markers by far predominate.
Greater numbers of DNA-markers can be identified to cover all regions of an organism’s DNA, and they are not based on the developmental stage of the plant as many protein-based markers are.
DNA-based markers can be derived from seeds or seedlings in rapid screening tests performed by automated robotic systems.
Plants lacking the desired traits can be eliminated before moving on to more expensive or lengthy greenhouse or field trials.
Additional resources:
|
Phenomics |
While the term genomics refers to the characterization of the DNA constitution of an organism, the term phenomics refers to the characterization of the phenotypic, or observable, traits of the organism.
Since genes can be recessive or dominant, whether an organism visibly exhibits a trait depends upon which types of genes it inherited from its parents.
When an organism has one gene (allele) that is recessive and one that is dominant for the same trait, only the dominate trait will be visible in the final form, or phenotype.
While genotyping can be performed at early stages of plant growth using only DNA, phenotypes generally require observation of the actual plant growth stage of interest.
With recent advances in genotyping technology, it has become much easier to determine a plant’s genotype than its phenotype.
Thus, there is intense interest in phenomics, which refers to the development of efficient, high-throughput methods to determine phenotypes of many individuals for multiple traits.
This extends not only to visible traits such as leaf shape or fruit color, but also to biochemical or metabolic traits that may be of interest.
|
Proteomics |
In general, the DNA in genes codes for the manufacture of proteins by the cellular protein synthesizing machinery.
Genes encoded by the DNA are converted into proteins selectively during development, such that only a subset of genes is being actively transcribed (read into messenger RNA molecules) and translated (converted into proteins) at any given time.
Thus, while an individual organism’s genotype is more or less fixed and constant in all of its cells, the proteome, or sum of all the proteins currently present, varies by cell type, developmental stage, and environmental conditions.
Proteomics is the study of the proteins that are produced by an organism or cell under various conditions.
Since tens of thousands of different proteins can be produced by an organism, proteomics employs techniques to first separate and then identify each of these proteins in an extracted sample.
Information about the proteins that are present can be linked with metabolic pathways to shed light on the types of processes that are occurring in the cells and to regulation of the genes that encode the proteins. |
|
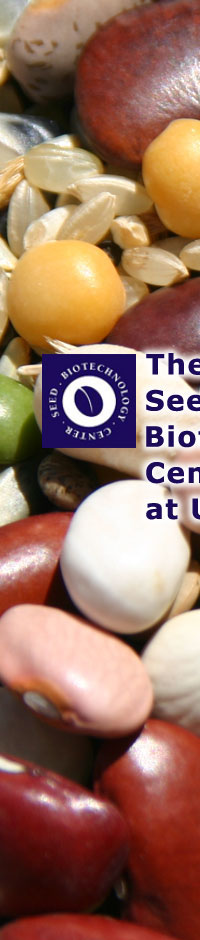
|
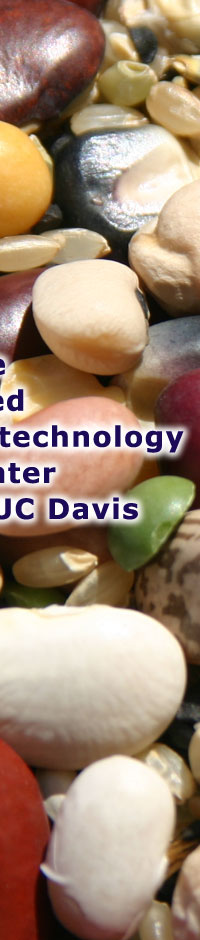
|